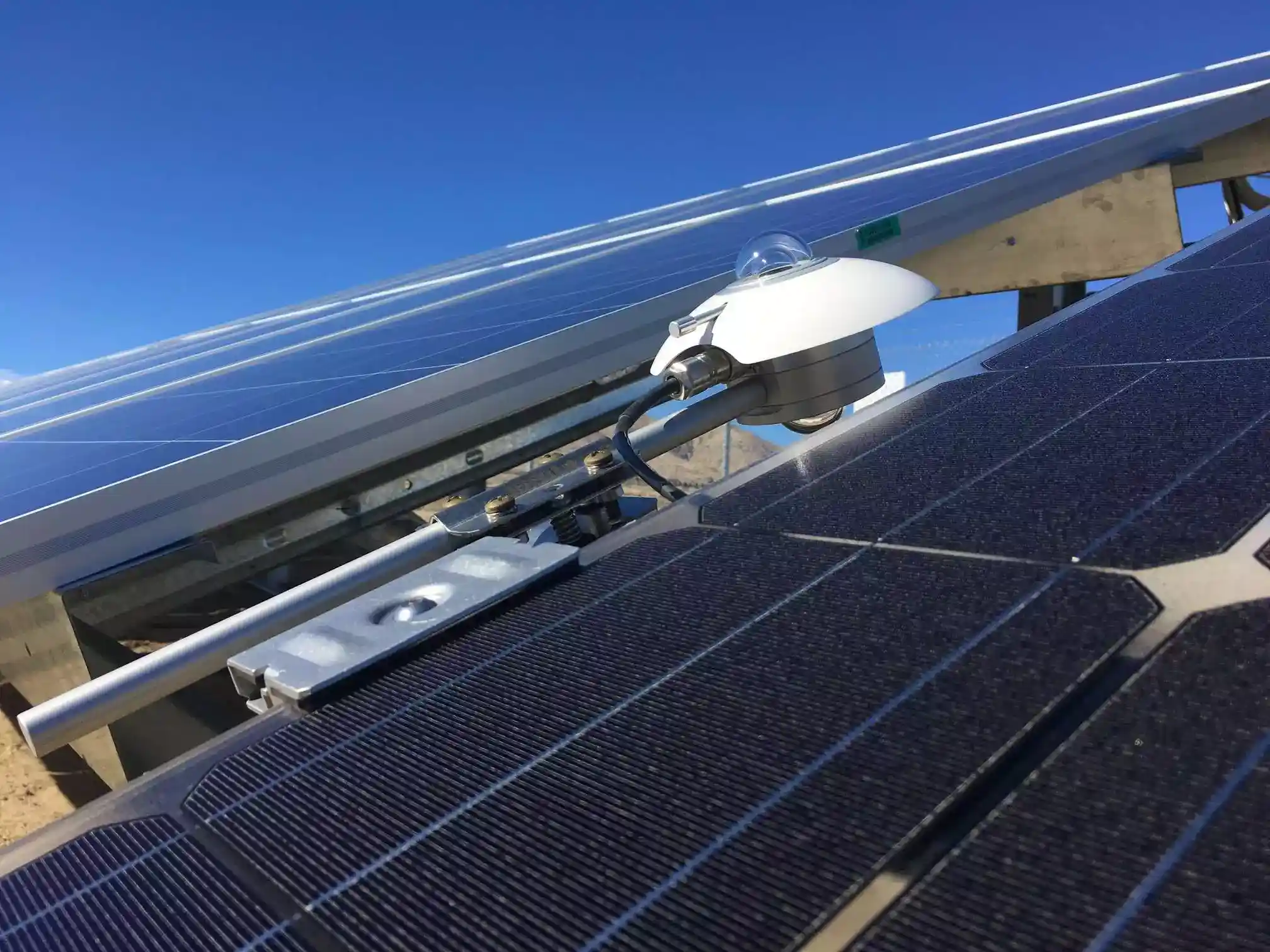
What is a Pyranometer?
Table of Contents
- How Pyraonometers Work
- Different Types of Pyranometers
- Applications of Pyranometers
- Advantages and Disadvantages of Using Pyranometers
- Calibrating Pyranometers
- Accuracy and Precision of Pyranometers
- Standards and Guidelines for Using Pyranometers
- Maintaining and Storing Pyranometers
- Environmental Effects on Pyranometer Measurements
- Cost and Lead Time for Purchasing Pyranometers
Pyranometers: A Comprehensive Guide for Measuring Solar Radiation
Pyranometers are devices that measure the amount of solar radiation, or sunlight, that is received by a surface. They are commonly used in a variety of applications, including solar energy production, meteorology, agriculture, and global energy balance studies for the purpose of global climate change research. In this article, we will cover everything you need to know about pyranometers, including how they work, different types available, applications, advantages and disadvantages, calibration, accuracy and precision, standards and guidelines, maintenance and storage, environmental effects, cost, and lead time.
How Pyranometers Work
Pyranometers are designed to measure the total amount of solar radiation received by a surface, commonly total hemispheric global solar radiation. They consist of a detector element, which is housed under an optic, hemispheric domes or diffuser, or a combination of both depending on the make and model. The pyranometer optic serves as a filter, transmitting the various shortwave solar wavelengths, from 285 – 3000 nm nominal, while rejecting far infrared wavelengths. Pyranometers employ one of two detector technologies: 1.) a silicon photodiode (photocell) detector which is typically housed an acrylic diffuser, or 2.) a blackbody thermopile detector which is housed under a glass dome, or a pair of glass domes. Silicon photodiode type pyranometers are lower cost devices which are limited in measurement accuracy due their limited spectral response range and spectral selectivity effect under changing spectral conditions.
Thermopile type pyranometers while usually higher in cost, respond to the true solar spectrum and do not suffer from spectral selectivity bias effect, and considered higher accuracy devices. The detector element converts any shortwave solar radiation received to an electrical signal (voltage or current), which is then sampled by the datalogger or metering device the pyranometer is connected to. The manufacturer supplies the instrument with a calibration report stating the sensitivity of the pyranometer, typically expressed in µV/(W/m²) units. The datalogger computes the irradiance in W/m² by dividing the sampled output signal of the pyranometer by the manufacturer supplied sensitivity value.
The industry governing standard by which pyranometers are rated in terms of performance, is the ISO 9060:2018 standard. The latest revision of the standard comprises three discrete performance classes: ISO 9060:2018 Class-A, Class-B, and Class-C.
- ISO 9060:2018 Class-C type pyranometers are the most economical and typically employed in routine measurement applications where absolute measurement accuracy is not a determining factor. Class-C pyranometers are inclusive of low-cost single dome spectrally flat thermopile type pyranometers, and lower costing acrylic diffuser type photoelectric type pyranometers which are not spectrally flat and limited in spectral response range.
- ISO 9060:2018 Class-B type pyranometers are higher performance instruments by comparison to Class-C model pyranometers, are spectrally flat in response, and typically employ a double dome optic design, or an outer dome optic with inner quartz diffuser. The required performance criteria to meet Class-B is also tighter than required to meet Class-C requirements. Class-B pyranometers are commonly used is meteorological monitoring applications and positioned between Class-C and Class-A on price.
- ISO 9060:2018 Class-A is the highest pyranometer performance class. In addition to all Class-A model pyranometers being spectrally flat in response. The required performance criteria to meet Class-A is more stringent than Class-C and Class-B. Any manufacturer branding their pyranometer as Class-A compliant, is required to individually test and document the performance of the pyranometer in writing, to ensure the pyranometer fully meets Class-A performance. Class-A pyranometers are higher in cost and commonly deployed in utility scale solar power plants to monitor plant performance / efficiency, as well as global energy balance monitoring networks where the highest degree of measurement accuracy is required.
HuksefluxUSA has a great selection of pyranometers on their website.
Applications of Pyranometers
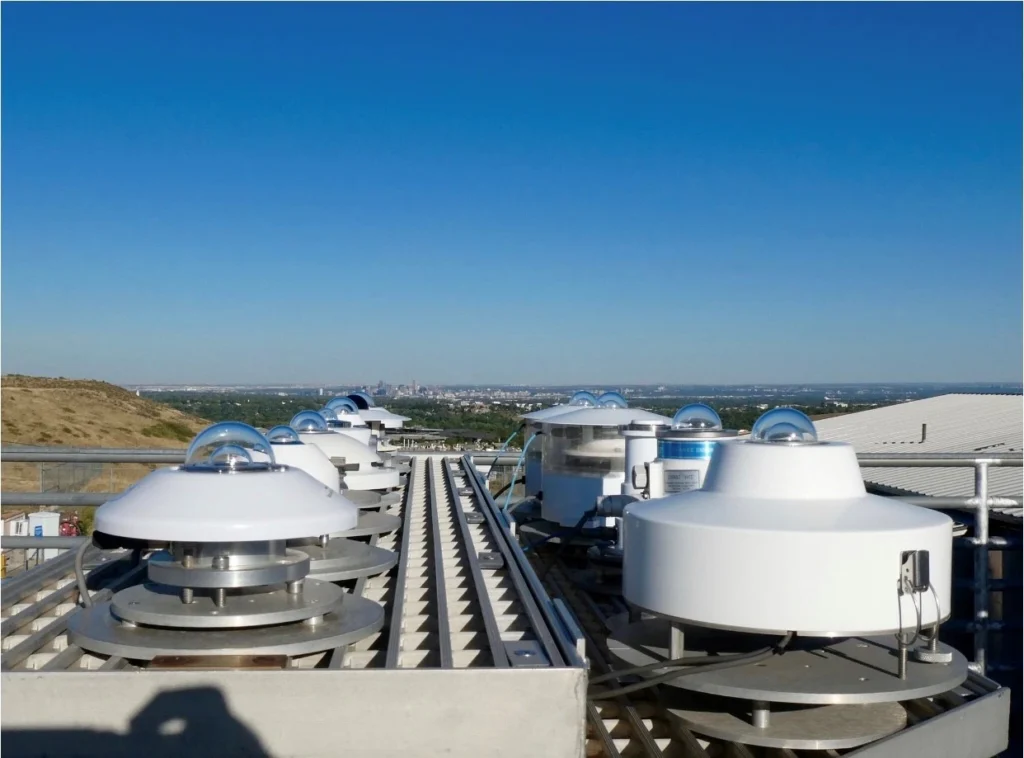
Pyranometers are used in a wide range of applications, including:
- Solar energy production: Pyranometers are used to measure the amount of solar radiation received by solar panels, which is essential for the efficient design and operation of solar power systems.
- Meteorology: Pyranometers are used in meteorological stations to measure the amount of solar radiation received by the Earth's surface, which is important for understanding weather patterns and climate.
- Agriculture: Pyranometers are used to measure the amount of solar radiation received by crops, which can help farmers optimize crop growth and yields.
- Other applications include monitoring air pollution, measuring UV radiation, and evaluating the performance of buildings and other structures.
Advantages and Disadvantages of Using Pyranometers
Pyranometers have many advantages, including:
- Solar energy production: Pyranometers are used to measure the amount of solar radiation received by solar panels, which is essential for PV system design and ongoing operation of any solar power plant, as plant PR (performance ratio) is quantifiable in real-time.
- Meteorology: Pyranometers are commonly installed on meteorological stations to measure the amount of incoming solar radiation received at the Earth's surface, including surface reflected solar radiation from the ground, both important parameters for understanding climate conditions and global shortwave energy balance.
- Agriculture: Pyranometers are used to measure the amount of solar radiation received by crops which can help farmers optimize crop growth and predict yield, assess conditions for crop disease, and evapotranspiration which can help crop growers optimize irrigation.
- Other applications include smart building automation, for optimizing energy efficiency and comfort.
However, there are also some disadvantages to using pyranometers, including:
- Cost: Pyranometers can be relatively expensive, especially for higher performance Class-A and Class-B spectrally flat thermopile models with advanced features.
- Maintenance: Pyranometers require routine maintenance, such as optics cleaning and calibration, to ensure accurate and reliable measurements. Required cleaning maintenance is site specific and varies from one site location to the next.
- Environmental effects: Pyranometer measurement accuracy can be adversely impacted by environmental factors, such as rain, ice, snow, and soiling. Ventilation and heating of the pyranometer, in addition to scheduled cleaning maintenance, greatly mitigates the potential for such measurement bias effects. For lower performance Class-C model pyranometers, ambient temperature extremes can also induce unwanted measurement bias effect due to inferior temperature response performance.
Calibrating Pyranometers
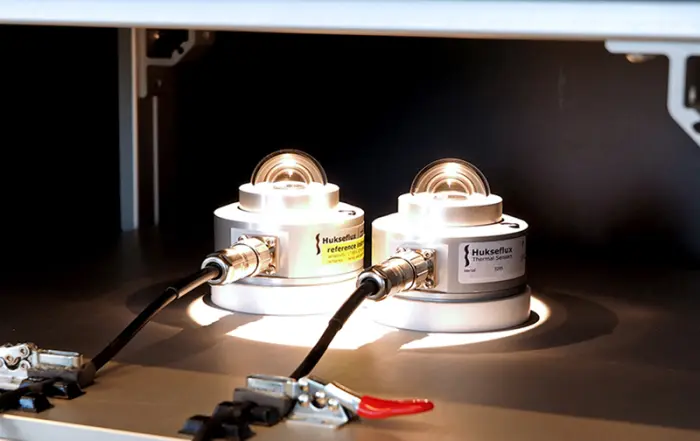
All pyranometers are subject to drift effect over time, commonly referred to as sensitivity drift. Drift effect varies amongst manufacturers depending on detector technology and optics construction, with spectrally flat Class-A model pyranometers exhibiting the lowest drift effect. At the time of production, the manufacturer determines the sensitivity of the pyranometer and documents the sensitivity value on the instrument calibration report. Often the expanded calibration uncertainty estimate associated with the reported sensitivity value is also stated on the manufacturer calibration report. Documenting pyranometer calibration uncertainty is important, as calibration uncertainty is a key input parameter for determining pyranometer measurement uncertainty at the field level according to the ASTM G213-17 measurement uncertainty standard: Standard Guide for Evaluating Uncertainty in Calibration and Field Measurements of Broadband Irradiance with Pyranometers and Pyrheliometers.
To ensure reliable pyranometer measurements, it is considered best practice to calibrate pyranometers on an annual or biannual basis, depending on user program requirements and the manufacturer’s recommended calibration interval. The sensitivity of all pyranometers is traceable to the WRR (World Radiometric Reference) which is a suite of primary reference absolute cavity pyrheliometers operated and maintained by PMOD-WRC laboratory, located in Davos Switzerland. There are multiple internationally recognized calibration standards (ISO and ASTM standards) for transferring the sensitivity of a primary or secondary reference radiometer, to a user pyranometer at the field level, or in a laboratory setting under controlled conditions. Most pyranometer manufacturers employ an indoors secondary transfer calibration method under an artificial sun (stabilized lamp source) to calibrate their production model pyranometers, in accordance with the ISO 9847 or ASTM G207-11 calibration standards.
The ISO 9847 and ASTM G207-11 indoors calibration standards are preferred by manufacturers as they result in lower (superior) expanded calibration uncertainty estimate vs. an outdoors secondary reference field calibration in accordance with the ISO 9847 or ASTM E824-10 calibration standards, which are otherwise subject to greater calibration uncertainty due to changing conditions outdoors at the time of calibration: e.g. sky conditions, solar zenith angle, spectral energy distribution, ambient temperature, induced thermal offset effects, etc.. An indoors ISO 9847 or ASTM G207-11 secondary transfer calibration conducted at normal incidence under a stabilized lamp source in a temperature-controlled laboratory environment, against a reference pyranometer of identical model type, minimizes the potential for such unwanted bias effects on the sensitivity transfer from the reference pyranometer to the user pyranometer, otherwise referred to the UUT (unit under test).
Accuracy and Precision of Pyranometers
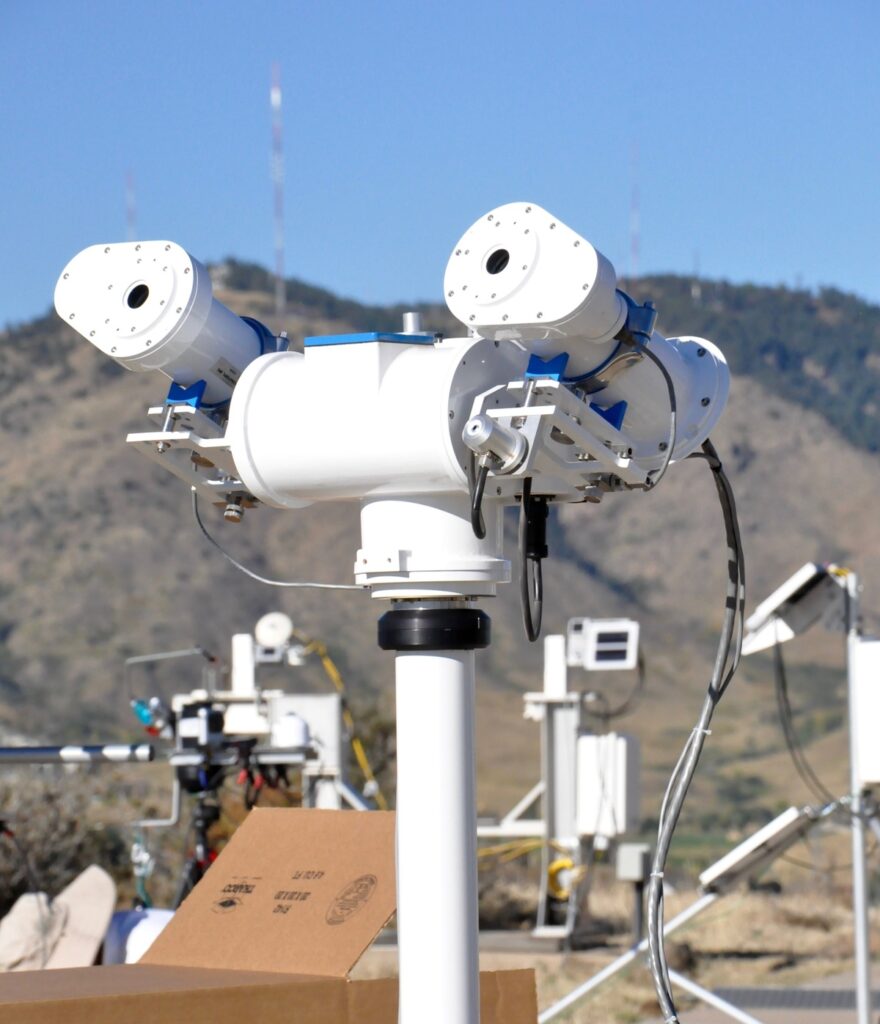
The accuracy and precision of pyranometers can vary depending on the model and the manufacturer. Measurement uncertainty at the field level will vary with pyranometer make and model type, and dependent on latitude and season. The best performing spectrally flat Class-A model pyranometers can achieve measurement uncertainties on total daily averages in ± 2.3% range with proper routine maintenance (mid-latitude, clear sky summer conditions), assuming a quality ISO 17025 accredited calibration with a documented expanded calibration uncertainty estimate of < ± 1% nominal. For a quality spectrally flat Class-B model pyranometer, measurement uncertainty estimates in the ± 7% range are achievable under the same conditions, while for Class-C model pyranometers measurement uncertainty estimates in the ± 14% range are achievable under the same conditions.
The lowest expanded calibration uncertainty estimates achievable for any given pyranometer make and model, can be accomplished by performing an outdoors primary reference transfer calibration against WRR traceable absolute cavity pyrheliometer in accordance with the ISO 9846 and ASTM G167-15 calibration standards. See image above of side-by-side primary reference absolute cavity pyrheliometers mounted on a two-axis solar tracker.
Standards and Guidelines for Using Pyranometers
There are several standards and guidelines that apply to the use of pyranometers, including:
- ISO 9060: This standard establishes the requirements for the design, performance, and testing of pyranometers.
- WMO Guide to Meteorological Instruments and Methods of Observation: This guide provides recommendations for the use of pyranometers in meteorological applications.
- ASTM G173-03: This standard provides the spectral irradiance data for the direct normal and hemispherical solar radiation.
There are other calibration standards. Read about all of them on our Calibration Standards page.
Maintaining and Storing Pyranometers
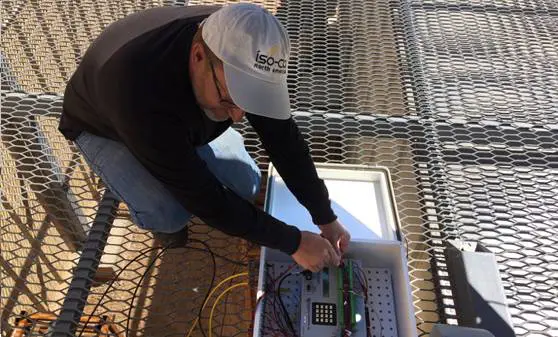
To ensure accurate and reliable pyranometer measurements, it is important to properly maintain and store pyranometers when not in use. This involves regular cleaning of the pyranometer optic and housing as needed, routine calibration per the manufacturer recommendation, and other maintenance checks such as pyranometer cable inspection, instrument leveling, and desiccant agent replacement as required. Pyranometers should be stored indoors in a climate-controlled facility, preferably at low to moderate year-round humidity levels.
Environmental Effects on Pyranometer Measurements
Pyranometer measurements can be affected by a variety of environmental factors, including:
- Temperature: The ambient temperature environment can impact pyranometer performance, so it is important to ensure the pyranometer is operating within the manufacturer's recommended temperature range. Temperature response performance will vary with ISO 9060:2018 performance class.
- Condensation: Higher humidity levels can result in condensation on the sensor optic depending on instrument surface temperature and dew point conditions. Condensation on the sensor optics will alter the normal transmission of light, resulting in substantial error bias effect. Pyranometers equipped with ventilation and heating will typically mitigate such adverse dew or frost deposition effects on the pyranometer optic.
- Dust and other contaminants: Dust and other contaminants can accumulate on the pyranometer optic which can adversely impact the pyranometer measurement performance. Proper routine scheduled maintenance that includes pyranometer optics cleaning, can greatly mitigate unwanted measurement bias effect due to optics soiling.
- Drift: Prolonged exposure to shortwave solar radiation in combination with year-round diurnal temperature cycling effect over time, can result in sensitivity drift. Biannual pyranometer calibration performed by an ISO 17025 accredited calibration laboratory is recommend by most manufacturers, to ensure optimal measure performance over the life of the sensor.
Cost and Lead Time for Purchasing Pyranometers
The cost of pyranometers can vary significantly depending on instrument make and model, standard features, and ISO 9060:2018 performance class. Class-C model pyranometers can cost as little as a few hundred dollars, while high-performance spectrally flat Class-A models with advanced features can cost several thousand dollars. Pyranometer delivery lead-times can vary as well depending on the instrument manufacturer and pyranometer model type. The market leader of thermopile type pyranometers is Hukseflux. The North America distributer HuksefluxUSA, offers great delivery lead-times and excellent local product support. Contact them through their website.
Conclusion
In conclusion, pyranometers are devices that measure the amount of solar radiation, or sunlight, received by surface the pyranometer is mounted on. They are commonly used in a variety of applications, including solar energy production, meteorology, and agriculture. This article covered the most important aspects of pyranometers, including how they work, detector technology and pyranometer performance class, applications, advantages and disadvantages, calibration, measurement uncertainty, standards and guidelines, maintenance and storage, environmental effects, instrument cost and lead-times.